What does vector operator for angular momentum measure?
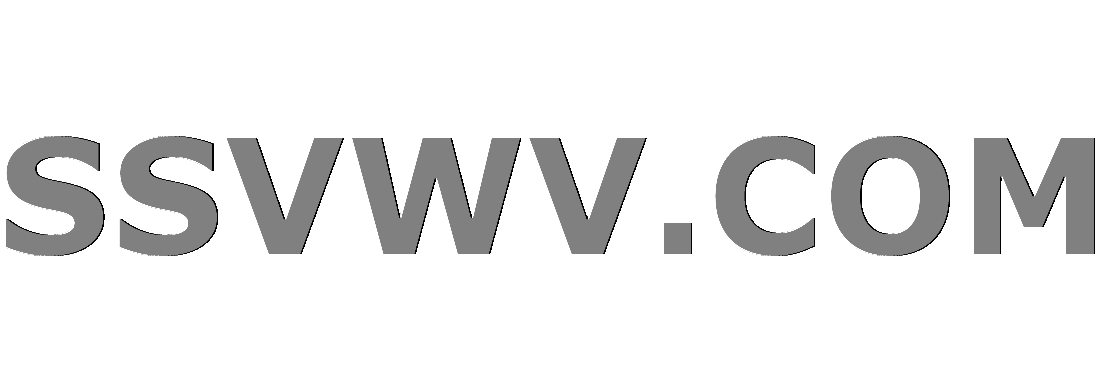
Multi tool use
$begingroup$
Consider the vector operator for angular momentum $hat L=hat L_x vec i +hat L_y vec j + hat L_z vec k$.
Does this mean that if we want to measure the angular momentum of a particle in state $psi$, we take $hat L$ and let it act on $psi$ to give us three possible eigenvalues of $hat L_x$, $hat L_y$ and $hat L_z$, which will correspond to the $x,y,z$ components of the angular momentum?
But since $hat L_x$, $hat L_y$ and $hat L_z$ does not commute, this should not be the meaning of $hat L$ because if we first make a measurement of $hat L_x$, the state of the particle will be changed, and $hat L_y$ should no longer act on the original state $psi$. What then does the vector operator $hat L$ gives us? More precisely, what is the measurement this operator $hat L$ is trying to measure?
quantum-mechanics angular-momentum operators vectors observables
$endgroup$
add a comment |
$begingroup$
Consider the vector operator for angular momentum $hat L=hat L_x vec i +hat L_y vec j + hat L_z vec k$.
Does this mean that if we want to measure the angular momentum of a particle in state $psi$, we take $hat L$ and let it act on $psi$ to give us three possible eigenvalues of $hat L_x$, $hat L_y$ and $hat L_z$, which will correspond to the $x,y,z$ components of the angular momentum?
But since $hat L_x$, $hat L_y$ and $hat L_z$ does not commute, this should not be the meaning of $hat L$ because if we first make a measurement of $hat L_x$, the state of the particle will be changed, and $hat L_y$ should no longer act on the original state $psi$. What then does the vector operator $hat L$ gives us? More precisely, what is the measurement this operator $hat L$ is trying to measure?
quantum-mechanics angular-momentum operators vectors observables
$endgroup$
add a comment |
$begingroup$
Consider the vector operator for angular momentum $hat L=hat L_x vec i +hat L_y vec j + hat L_z vec k$.
Does this mean that if we want to measure the angular momentum of a particle in state $psi$, we take $hat L$ and let it act on $psi$ to give us three possible eigenvalues of $hat L_x$, $hat L_y$ and $hat L_z$, which will correspond to the $x,y,z$ components of the angular momentum?
But since $hat L_x$, $hat L_y$ and $hat L_z$ does not commute, this should not be the meaning of $hat L$ because if we first make a measurement of $hat L_x$, the state of the particle will be changed, and $hat L_y$ should no longer act on the original state $psi$. What then does the vector operator $hat L$ gives us? More precisely, what is the measurement this operator $hat L$ is trying to measure?
quantum-mechanics angular-momentum operators vectors observables
$endgroup$
Consider the vector operator for angular momentum $hat L=hat L_x vec i +hat L_y vec j + hat L_z vec k$.
Does this mean that if we want to measure the angular momentum of a particle in state $psi$, we take $hat L$ and let it act on $psi$ to give us three possible eigenvalues of $hat L_x$, $hat L_y$ and $hat L_z$, which will correspond to the $x,y,z$ components of the angular momentum?
But since $hat L_x$, $hat L_y$ and $hat L_z$ does not commute, this should not be the meaning of $hat L$ because if we first make a measurement of $hat L_x$, the state of the particle will be changed, and $hat L_y$ should no longer act on the original state $psi$. What then does the vector operator $hat L$ gives us? More precisely, what is the measurement this operator $hat L$ is trying to measure?
quantum-mechanics angular-momentum operators vectors observables
quantum-mechanics angular-momentum operators vectors observables
edited Dec 20 '18 at 17:34
TaeNyFan
asked Dec 20 '18 at 17:13


TaeNyFanTaeNyFan
3077
3077
add a comment |
add a comment |
6 Answers
6
active
oldest
votes
$begingroup$
The fact that $hat L_x$, $hat L_y$ and $hat L_z$ do not commute means that you cannot have a function which is eigenfunction of the three operators. Your function can only be an eigenfunction of one of the component operators, say $hat L_z$ (it is the typically chosen one). However, the operator $hat L^2$ does commute with each of the component operators. So if we have an eigenfunction of $hat L_z$, it is also an eigenfunction of $hat L^2$. (But not an eigenfunction of $hat L_x$ and $hat L_y$).
Therefore, the answer to your question is that the operator $hat L$ is used to give us the magnitude of the angular momentum, by operating on the eigenfunction with its square: $hat L^2$. The eigenvalue is then $l(l+1)ħ^2$, which is the magnitude of the angular momentum squared.
$endgroup$
add a comment |
$begingroup$
You can only measure operators. A vector operator is a set of three operators, which we package into one piece of notation because they transform under rotations in a nice way. You can't measure it all in one go, any more than you can try to measure $hat{x}$ and $hat{p}$ at the same time. However, you can measure the components of $hat{L}$, which are, as you would expect, the components of the angular momentum.
$endgroup$
$begingroup$
Also, aside from transformation under rotations, using this not-fully-measurable vector "operator" also lets us build other operators corresponding to such quantities as square of angular momentum $L^2$ or Laplace-Runge-Lenz vector $mathbf A=mkfrac{mathbf{r}}r-frac12(mathbf{p}timesmathbf{L}-mathbf{L}timesmathbf{p})$.
$endgroup$
– Ruslan
Dec 21 '18 at 7:15
add a comment |
$begingroup$
I like this question. What you describe is taking:
$${bf hat L}|lrangle = hat L_x|lrangle {bf hat x} + hat L_y hat L_x|lrangle {bf hat y} ... = {bf vec L}|lrangle$$
which would lead to problems. I think an eigenvalue equation for a vector operator should be:
$${bf hat L}|lrangle = hat L_x|lrangle {bf hat x} + hat L_y|lrangle {bf hat y} + hat L_z|lrangle {bf hat z} = L_x|lrangle {bf hat x} + L_y|lrangle {bf hat y} + L_z|lrangle {bf hat z} = {bf vec L}|l>$$
where
$${bf hat L} = -ihbar (yfrac{partial}{partial z} - zfrac{partial}{partial y}){bf hat x} + (permutations) $$
is the operator and
$${bf vec L} = L_x {bf hat x}+ L_y {bf hat y} + L_z {bf hat z }$$
is an ordinary vector.
Now with:
$$ |lrangle equiv F_l(x, y, z)$$
the equation:
$$ {bf hat L}F_l(x, y, z) = {bf vec L}F_l(x, y, z)$$
doesn't have any non-trivial solutions: there are no eigenstates of completely known (non zero) angular momentum.
Regarding your question on "meaning": note that:
$$ L_z = -ihbarfrac{partial}{partialphi} $$
and eigenstates of that function look like:
$$ F(r, theta, phi) = R(r)Theta(theta)e^{pm i m phi} $$
which means that the function is invariant under rotations of $2pi/m$.
For a wave-function to be an eigenstate of ${bf hat L}$ it would have to invariant under some non-trivial rotation about not just the $z$-axis, but the $x$ and $y$ axes also--which would mean it would have to be invariant under some rotation about any axis.
The only thing I can think of that can do that is a sphere, and this is, of course, the zero angular momentum eigenstate.
$endgroup$
add a comment |
$begingroup$
Basically you are asking does a state exist which is a simultaneous eigenstate of the three components. The answer is no. You can construct a state that is an eigenfunction of $L^2$ and any one component, usually the z-component is chosen. This is due to the commutation relations. If a state exists whose all three components are known then a rotation about any three axis will not change the state. But this would mean that the state would be spherically symmetric which is only possible if $L = 0$.
$endgroup$
add a comment |
$begingroup$
Does this mean that if we want to measure the angular momentum of a particle in state $ψ$, we take $hat L$ and let it act on $ψ$ to give us three possible eigenvalues of $hat L_x$, $hat L_y$ and $hat L_z$, which will correspond to the x,y,z components of the angular momentum?
As you know $L_x$, $L_y$ and $L_z$ are incompatible observables, but note that $L^2$ does commute with the three components of the angular momentum, so:
$$[L^2, Lx] = [L^2, Ly] =[L^2, Lz]=0$$
Which more compactly is:
$$[L^2, L] = 0$$
Based on it, it is feasible to find simultaneous eigenstates of $L^2$ and one of the three components of the angular momentum. Using as an example $L_z$ we would get:
$$L^2 f = lambda f$$
$$L_z f = lambda' f$$
What is the measurement this operator $hat L$ is trying to measure?
Because of compatibility reasons, $L^2$ and the components of the angular momentum are worked out separately.
Actually, to know $hat L$ you should know simultaneously the three components of the angular momentum which is not the case. The uncertainty principle denies it.
$endgroup$
add a comment |
$begingroup$
This is right. You cannot have an eigenstate and eigenvalue of this vectorial angular momentum operator $hat{mathbf{L}}$. In fact, one doesn't even need the commutation to see that this cannot work. The reasons are purely mathematical. Just consider that given an operator $hat{O}$, its eigenvalue equation is
$$hat{O} |psirangle = O |psirangle$$
where $O$ is the eigenvalue, which is generally a complex number, but should be a real number for operators representing physical parameters. Now consider what this should look like for vectorial operator $hat{mathbf{L}}$. We should, following the same logic, have it associated with an eigenvalue that is itself a vector, representing a specific angular momentum vector $mathbf{L}$, giving
$$hat{mathbf{L}} |psirangle = mathbf{L} |psirangle$$
But look at the right hand side. We have a vector multiplying a quantum state. That's not mathematically possible. You can only multiply a quantum state by a complex scalar, because that's the only other operation than addition and taking the inner product that is defined on the Hilbert space. So by that token alone, $hat{mathbf{L}}$ cannot have "eigenvalues" in this sense. And this makes sense: the output of applying your $hat{mathbf{L}}$ to a quantum state isn't even another quantum state, but instead the "vector of quantum states" (a "meta-vector", perhaps?)
$$hat{mathbf{L}} |psirangle = (hat{L}_x |psirangle) mathbf{i} + (hat{L}_y |psirangle) mathbf{j} + (hat{L}_z |psirangle) mathbf{k}$$
Thus even worse, technically your proposed "operator" isn't really a proper operator at all, but a map between two rather different vector spaces, and no such maps can have eigenvalues, only self-maps of the same vector space.
$endgroup$
add a comment |
Your Answer
StackExchange.ifUsing("editor", function () {
return StackExchange.using("mathjaxEditing", function () {
StackExchange.MarkdownEditor.creationCallbacks.add(function (editor, postfix) {
StackExchange.mathjaxEditing.prepareWmdForMathJax(editor, postfix, [["$", "$"], ["\\(","\\)"]]);
});
});
}, "mathjax-editing");
StackExchange.ready(function() {
var channelOptions = {
tags: "".split(" "),
id: "151"
};
initTagRenderer("".split(" "), "".split(" "), channelOptions);
StackExchange.using("externalEditor", function() {
// Have to fire editor after snippets, if snippets enabled
if (StackExchange.settings.snippets.snippetsEnabled) {
StackExchange.using("snippets", function() {
createEditor();
});
}
else {
createEditor();
}
});
function createEditor() {
StackExchange.prepareEditor({
heartbeatType: 'answer',
autoActivateHeartbeat: false,
convertImagesToLinks: false,
noModals: true,
showLowRepImageUploadWarning: true,
reputationToPostImages: null,
bindNavPrevention: true,
postfix: "",
imageUploader: {
brandingHtml: "Powered by u003ca class="icon-imgur-white" href="https://imgur.com/"u003eu003c/au003e",
contentPolicyHtml: "User contributions licensed under u003ca href="https://creativecommons.org/licenses/by-sa/3.0/"u003ecc by-sa 3.0 with attribution requiredu003c/au003e u003ca href="https://stackoverflow.com/legal/content-policy"u003e(content policy)u003c/au003e",
allowUrls: true
},
noCode: true, onDemand: true,
discardSelector: ".discard-answer"
,immediatelyShowMarkdownHelp:true
});
}
});
Sign up or log in
StackExchange.ready(function () {
StackExchange.helpers.onClickDraftSave('#login-link');
});
Sign up using Google
Sign up using Facebook
Sign up using Email and Password
Post as a guest
Required, but never shown
StackExchange.ready(
function () {
StackExchange.openid.initPostLogin('.new-post-login', 'https%3a%2f%2fphysics.stackexchange.com%2fquestions%2f449543%2fwhat-does-vector-operator-for-angular-momentum-measure%23new-answer', 'question_page');
}
);
Post as a guest
Required, but never shown
6 Answers
6
active
oldest
votes
6 Answers
6
active
oldest
votes
active
oldest
votes
active
oldest
votes
$begingroup$
The fact that $hat L_x$, $hat L_y$ and $hat L_z$ do not commute means that you cannot have a function which is eigenfunction of the three operators. Your function can only be an eigenfunction of one of the component operators, say $hat L_z$ (it is the typically chosen one). However, the operator $hat L^2$ does commute with each of the component operators. So if we have an eigenfunction of $hat L_z$, it is also an eigenfunction of $hat L^2$. (But not an eigenfunction of $hat L_x$ and $hat L_y$).
Therefore, the answer to your question is that the operator $hat L$ is used to give us the magnitude of the angular momentum, by operating on the eigenfunction with its square: $hat L^2$. The eigenvalue is then $l(l+1)ħ^2$, which is the magnitude of the angular momentum squared.
$endgroup$
add a comment |
$begingroup$
The fact that $hat L_x$, $hat L_y$ and $hat L_z$ do not commute means that you cannot have a function which is eigenfunction of the three operators. Your function can only be an eigenfunction of one of the component operators, say $hat L_z$ (it is the typically chosen one). However, the operator $hat L^2$ does commute with each of the component operators. So if we have an eigenfunction of $hat L_z$, it is also an eigenfunction of $hat L^2$. (But not an eigenfunction of $hat L_x$ and $hat L_y$).
Therefore, the answer to your question is that the operator $hat L$ is used to give us the magnitude of the angular momentum, by operating on the eigenfunction with its square: $hat L^2$. The eigenvalue is then $l(l+1)ħ^2$, which is the magnitude of the angular momentum squared.
$endgroup$
add a comment |
$begingroup$
The fact that $hat L_x$, $hat L_y$ and $hat L_z$ do not commute means that you cannot have a function which is eigenfunction of the three operators. Your function can only be an eigenfunction of one of the component operators, say $hat L_z$ (it is the typically chosen one). However, the operator $hat L^2$ does commute with each of the component operators. So if we have an eigenfunction of $hat L_z$, it is also an eigenfunction of $hat L^2$. (But not an eigenfunction of $hat L_x$ and $hat L_y$).
Therefore, the answer to your question is that the operator $hat L$ is used to give us the magnitude of the angular momentum, by operating on the eigenfunction with its square: $hat L^2$. The eigenvalue is then $l(l+1)ħ^2$, which is the magnitude of the angular momentum squared.
$endgroup$
The fact that $hat L_x$, $hat L_y$ and $hat L_z$ do not commute means that you cannot have a function which is eigenfunction of the three operators. Your function can only be an eigenfunction of one of the component operators, say $hat L_z$ (it is the typically chosen one). However, the operator $hat L^2$ does commute with each of the component operators. So if we have an eigenfunction of $hat L_z$, it is also an eigenfunction of $hat L^2$. (But not an eigenfunction of $hat L_x$ and $hat L_y$).
Therefore, the answer to your question is that the operator $hat L$ is used to give us the magnitude of the angular momentum, by operating on the eigenfunction with its square: $hat L^2$. The eigenvalue is then $l(l+1)ħ^2$, which is the magnitude of the angular momentum squared.
edited Dec 20 '18 at 23:56
answered Dec 20 '18 at 17:45


Luismi98Luismi98
12810
12810
add a comment |
add a comment |
$begingroup$
You can only measure operators. A vector operator is a set of three operators, which we package into one piece of notation because they transform under rotations in a nice way. You can't measure it all in one go, any more than you can try to measure $hat{x}$ and $hat{p}$ at the same time. However, you can measure the components of $hat{L}$, which are, as you would expect, the components of the angular momentum.
$endgroup$
$begingroup$
Also, aside from transformation under rotations, using this not-fully-measurable vector "operator" also lets us build other operators corresponding to such quantities as square of angular momentum $L^2$ or Laplace-Runge-Lenz vector $mathbf A=mkfrac{mathbf{r}}r-frac12(mathbf{p}timesmathbf{L}-mathbf{L}timesmathbf{p})$.
$endgroup$
– Ruslan
Dec 21 '18 at 7:15
add a comment |
$begingroup$
You can only measure operators. A vector operator is a set of three operators, which we package into one piece of notation because they transform under rotations in a nice way. You can't measure it all in one go, any more than you can try to measure $hat{x}$ and $hat{p}$ at the same time. However, you can measure the components of $hat{L}$, which are, as you would expect, the components of the angular momentum.
$endgroup$
$begingroup$
Also, aside from transformation under rotations, using this not-fully-measurable vector "operator" also lets us build other operators corresponding to such quantities as square of angular momentum $L^2$ or Laplace-Runge-Lenz vector $mathbf A=mkfrac{mathbf{r}}r-frac12(mathbf{p}timesmathbf{L}-mathbf{L}timesmathbf{p})$.
$endgroup$
– Ruslan
Dec 21 '18 at 7:15
add a comment |
$begingroup$
You can only measure operators. A vector operator is a set of three operators, which we package into one piece of notation because they transform under rotations in a nice way. You can't measure it all in one go, any more than you can try to measure $hat{x}$ and $hat{p}$ at the same time. However, you can measure the components of $hat{L}$, which are, as you would expect, the components of the angular momentum.
$endgroup$
You can only measure operators. A vector operator is a set of three operators, which we package into one piece of notation because they transform under rotations in a nice way. You can't measure it all in one go, any more than you can try to measure $hat{x}$ and $hat{p}$ at the same time. However, you can measure the components of $hat{L}$, which are, as you would expect, the components of the angular momentum.
answered Dec 20 '18 at 17:43
knzhouknzhou
43.5k11118207
43.5k11118207
$begingroup$
Also, aside from transformation under rotations, using this not-fully-measurable vector "operator" also lets us build other operators corresponding to such quantities as square of angular momentum $L^2$ or Laplace-Runge-Lenz vector $mathbf A=mkfrac{mathbf{r}}r-frac12(mathbf{p}timesmathbf{L}-mathbf{L}timesmathbf{p})$.
$endgroup$
– Ruslan
Dec 21 '18 at 7:15
add a comment |
$begingroup$
Also, aside from transformation under rotations, using this not-fully-measurable vector "operator" also lets us build other operators corresponding to such quantities as square of angular momentum $L^2$ or Laplace-Runge-Lenz vector $mathbf A=mkfrac{mathbf{r}}r-frac12(mathbf{p}timesmathbf{L}-mathbf{L}timesmathbf{p})$.
$endgroup$
– Ruslan
Dec 21 '18 at 7:15
$begingroup$
Also, aside from transformation under rotations, using this not-fully-measurable vector "operator" also lets us build other operators corresponding to such quantities as square of angular momentum $L^2$ or Laplace-Runge-Lenz vector $mathbf A=mkfrac{mathbf{r}}r-frac12(mathbf{p}timesmathbf{L}-mathbf{L}timesmathbf{p})$.
$endgroup$
– Ruslan
Dec 21 '18 at 7:15
$begingroup$
Also, aside from transformation under rotations, using this not-fully-measurable vector "operator" also lets us build other operators corresponding to such quantities as square of angular momentum $L^2$ or Laplace-Runge-Lenz vector $mathbf A=mkfrac{mathbf{r}}r-frac12(mathbf{p}timesmathbf{L}-mathbf{L}timesmathbf{p})$.
$endgroup$
– Ruslan
Dec 21 '18 at 7:15
add a comment |
$begingroup$
I like this question. What you describe is taking:
$${bf hat L}|lrangle = hat L_x|lrangle {bf hat x} + hat L_y hat L_x|lrangle {bf hat y} ... = {bf vec L}|lrangle$$
which would lead to problems. I think an eigenvalue equation for a vector operator should be:
$${bf hat L}|lrangle = hat L_x|lrangle {bf hat x} + hat L_y|lrangle {bf hat y} + hat L_z|lrangle {bf hat z} = L_x|lrangle {bf hat x} + L_y|lrangle {bf hat y} + L_z|lrangle {bf hat z} = {bf vec L}|l>$$
where
$${bf hat L} = -ihbar (yfrac{partial}{partial z} - zfrac{partial}{partial y}){bf hat x} + (permutations) $$
is the operator and
$${bf vec L} = L_x {bf hat x}+ L_y {bf hat y} + L_z {bf hat z }$$
is an ordinary vector.
Now with:
$$ |lrangle equiv F_l(x, y, z)$$
the equation:
$$ {bf hat L}F_l(x, y, z) = {bf vec L}F_l(x, y, z)$$
doesn't have any non-trivial solutions: there are no eigenstates of completely known (non zero) angular momentum.
Regarding your question on "meaning": note that:
$$ L_z = -ihbarfrac{partial}{partialphi} $$
and eigenstates of that function look like:
$$ F(r, theta, phi) = R(r)Theta(theta)e^{pm i m phi} $$
which means that the function is invariant under rotations of $2pi/m$.
For a wave-function to be an eigenstate of ${bf hat L}$ it would have to invariant under some non-trivial rotation about not just the $z$-axis, but the $x$ and $y$ axes also--which would mean it would have to be invariant under some rotation about any axis.
The only thing I can think of that can do that is a sphere, and this is, of course, the zero angular momentum eigenstate.
$endgroup$
add a comment |
$begingroup$
I like this question. What you describe is taking:
$${bf hat L}|lrangle = hat L_x|lrangle {bf hat x} + hat L_y hat L_x|lrangle {bf hat y} ... = {bf vec L}|lrangle$$
which would lead to problems. I think an eigenvalue equation for a vector operator should be:
$${bf hat L}|lrangle = hat L_x|lrangle {bf hat x} + hat L_y|lrangle {bf hat y} + hat L_z|lrangle {bf hat z} = L_x|lrangle {bf hat x} + L_y|lrangle {bf hat y} + L_z|lrangle {bf hat z} = {bf vec L}|l>$$
where
$${bf hat L} = -ihbar (yfrac{partial}{partial z} - zfrac{partial}{partial y}){bf hat x} + (permutations) $$
is the operator and
$${bf vec L} = L_x {bf hat x}+ L_y {bf hat y} + L_z {bf hat z }$$
is an ordinary vector.
Now with:
$$ |lrangle equiv F_l(x, y, z)$$
the equation:
$$ {bf hat L}F_l(x, y, z) = {bf vec L}F_l(x, y, z)$$
doesn't have any non-trivial solutions: there are no eigenstates of completely known (non zero) angular momentum.
Regarding your question on "meaning": note that:
$$ L_z = -ihbarfrac{partial}{partialphi} $$
and eigenstates of that function look like:
$$ F(r, theta, phi) = R(r)Theta(theta)e^{pm i m phi} $$
which means that the function is invariant under rotations of $2pi/m$.
For a wave-function to be an eigenstate of ${bf hat L}$ it would have to invariant under some non-trivial rotation about not just the $z$-axis, but the $x$ and $y$ axes also--which would mean it would have to be invariant under some rotation about any axis.
The only thing I can think of that can do that is a sphere, and this is, of course, the zero angular momentum eigenstate.
$endgroup$
add a comment |
$begingroup$
I like this question. What you describe is taking:
$${bf hat L}|lrangle = hat L_x|lrangle {bf hat x} + hat L_y hat L_x|lrangle {bf hat y} ... = {bf vec L}|lrangle$$
which would lead to problems. I think an eigenvalue equation for a vector operator should be:
$${bf hat L}|lrangle = hat L_x|lrangle {bf hat x} + hat L_y|lrangle {bf hat y} + hat L_z|lrangle {bf hat z} = L_x|lrangle {bf hat x} + L_y|lrangle {bf hat y} + L_z|lrangle {bf hat z} = {bf vec L}|l>$$
where
$${bf hat L} = -ihbar (yfrac{partial}{partial z} - zfrac{partial}{partial y}){bf hat x} + (permutations) $$
is the operator and
$${bf vec L} = L_x {bf hat x}+ L_y {bf hat y} + L_z {bf hat z }$$
is an ordinary vector.
Now with:
$$ |lrangle equiv F_l(x, y, z)$$
the equation:
$$ {bf hat L}F_l(x, y, z) = {bf vec L}F_l(x, y, z)$$
doesn't have any non-trivial solutions: there are no eigenstates of completely known (non zero) angular momentum.
Regarding your question on "meaning": note that:
$$ L_z = -ihbarfrac{partial}{partialphi} $$
and eigenstates of that function look like:
$$ F(r, theta, phi) = R(r)Theta(theta)e^{pm i m phi} $$
which means that the function is invariant under rotations of $2pi/m$.
For a wave-function to be an eigenstate of ${bf hat L}$ it would have to invariant under some non-trivial rotation about not just the $z$-axis, but the $x$ and $y$ axes also--which would mean it would have to be invariant under some rotation about any axis.
The only thing I can think of that can do that is a sphere, and this is, of course, the zero angular momentum eigenstate.
$endgroup$
I like this question. What you describe is taking:
$${bf hat L}|lrangle = hat L_x|lrangle {bf hat x} + hat L_y hat L_x|lrangle {bf hat y} ... = {bf vec L}|lrangle$$
which would lead to problems. I think an eigenvalue equation for a vector operator should be:
$${bf hat L}|lrangle = hat L_x|lrangle {bf hat x} + hat L_y|lrangle {bf hat y} + hat L_z|lrangle {bf hat z} = L_x|lrangle {bf hat x} + L_y|lrangle {bf hat y} + L_z|lrangle {bf hat z} = {bf vec L}|l>$$
where
$${bf hat L} = -ihbar (yfrac{partial}{partial z} - zfrac{partial}{partial y}){bf hat x} + (permutations) $$
is the operator and
$${bf vec L} = L_x {bf hat x}+ L_y {bf hat y} + L_z {bf hat z }$$
is an ordinary vector.
Now with:
$$ |lrangle equiv F_l(x, y, z)$$
the equation:
$$ {bf hat L}F_l(x, y, z) = {bf vec L}F_l(x, y, z)$$
doesn't have any non-trivial solutions: there are no eigenstates of completely known (non zero) angular momentum.
Regarding your question on "meaning": note that:
$$ L_z = -ihbarfrac{partial}{partialphi} $$
and eigenstates of that function look like:
$$ F(r, theta, phi) = R(r)Theta(theta)e^{pm i m phi} $$
which means that the function is invariant under rotations of $2pi/m$.
For a wave-function to be an eigenstate of ${bf hat L}$ it would have to invariant under some non-trivial rotation about not just the $z$-axis, but the $x$ and $y$ axes also--which would mean it would have to be invariant under some rotation about any axis.
The only thing I can think of that can do that is a sphere, and this is, of course, the zero angular momentum eigenstate.
edited Dec 21 '18 at 0:34
answered Dec 20 '18 at 17:39
JEBJEB
5,8831717
5,8831717
add a comment |
add a comment |
$begingroup$
Basically you are asking does a state exist which is a simultaneous eigenstate of the three components. The answer is no. You can construct a state that is an eigenfunction of $L^2$ and any one component, usually the z-component is chosen. This is due to the commutation relations. If a state exists whose all three components are known then a rotation about any three axis will not change the state. But this would mean that the state would be spherically symmetric which is only possible if $L = 0$.
$endgroup$
add a comment |
$begingroup$
Basically you are asking does a state exist which is a simultaneous eigenstate of the three components. The answer is no. You can construct a state that is an eigenfunction of $L^2$ and any one component, usually the z-component is chosen. This is due to the commutation relations. If a state exists whose all three components are known then a rotation about any three axis will not change the state. But this would mean that the state would be spherically symmetric which is only possible if $L = 0$.
$endgroup$
add a comment |
$begingroup$
Basically you are asking does a state exist which is a simultaneous eigenstate of the three components. The answer is no. You can construct a state that is an eigenfunction of $L^2$ and any one component, usually the z-component is chosen. This is due to the commutation relations. If a state exists whose all three components are known then a rotation about any three axis will not change the state. But this would mean that the state would be spherically symmetric which is only possible if $L = 0$.
$endgroup$
Basically you are asking does a state exist which is a simultaneous eigenstate of the three components. The answer is no. You can construct a state that is an eigenfunction of $L^2$ and any one component, usually the z-component is chosen. This is due to the commutation relations. If a state exists whose all three components are known then a rotation about any three axis will not change the state. But this would mean that the state would be spherically symmetric which is only possible if $L = 0$.
edited Dec 20 '18 at 18:45
JMac
8,54921832
8,54921832
answered Dec 20 '18 at 18:28
SAKhanSAKhan
1,057612
1,057612
add a comment |
add a comment |
$begingroup$
Does this mean that if we want to measure the angular momentum of a particle in state $ψ$, we take $hat L$ and let it act on $ψ$ to give us three possible eigenvalues of $hat L_x$, $hat L_y$ and $hat L_z$, which will correspond to the x,y,z components of the angular momentum?
As you know $L_x$, $L_y$ and $L_z$ are incompatible observables, but note that $L^2$ does commute with the three components of the angular momentum, so:
$$[L^2, Lx] = [L^2, Ly] =[L^2, Lz]=0$$
Which more compactly is:
$$[L^2, L] = 0$$
Based on it, it is feasible to find simultaneous eigenstates of $L^2$ and one of the three components of the angular momentum. Using as an example $L_z$ we would get:
$$L^2 f = lambda f$$
$$L_z f = lambda' f$$
What is the measurement this operator $hat L$ is trying to measure?
Because of compatibility reasons, $L^2$ and the components of the angular momentum are worked out separately.
Actually, to know $hat L$ you should know simultaneously the three components of the angular momentum which is not the case. The uncertainty principle denies it.
$endgroup$
add a comment |
$begingroup$
Does this mean that if we want to measure the angular momentum of a particle in state $ψ$, we take $hat L$ and let it act on $ψ$ to give us three possible eigenvalues of $hat L_x$, $hat L_y$ and $hat L_z$, which will correspond to the x,y,z components of the angular momentum?
As you know $L_x$, $L_y$ and $L_z$ are incompatible observables, but note that $L^2$ does commute with the three components of the angular momentum, so:
$$[L^2, Lx] = [L^2, Ly] =[L^2, Lz]=0$$
Which more compactly is:
$$[L^2, L] = 0$$
Based on it, it is feasible to find simultaneous eigenstates of $L^2$ and one of the three components of the angular momentum. Using as an example $L_z$ we would get:
$$L^2 f = lambda f$$
$$L_z f = lambda' f$$
What is the measurement this operator $hat L$ is trying to measure?
Because of compatibility reasons, $L^2$ and the components of the angular momentum are worked out separately.
Actually, to know $hat L$ you should know simultaneously the three components of the angular momentum which is not the case. The uncertainty principle denies it.
$endgroup$
add a comment |
$begingroup$
Does this mean that if we want to measure the angular momentum of a particle in state $ψ$, we take $hat L$ and let it act on $ψ$ to give us three possible eigenvalues of $hat L_x$, $hat L_y$ and $hat L_z$, which will correspond to the x,y,z components of the angular momentum?
As you know $L_x$, $L_y$ and $L_z$ are incompatible observables, but note that $L^2$ does commute with the three components of the angular momentum, so:
$$[L^2, Lx] = [L^2, Ly] =[L^2, Lz]=0$$
Which more compactly is:
$$[L^2, L] = 0$$
Based on it, it is feasible to find simultaneous eigenstates of $L^2$ and one of the three components of the angular momentum. Using as an example $L_z$ we would get:
$$L^2 f = lambda f$$
$$L_z f = lambda' f$$
What is the measurement this operator $hat L$ is trying to measure?
Because of compatibility reasons, $L^2$ and the components of the angular momentum are worked out separately.
Actually, to know $hat L$ you should know simultaneously the three components of the angular momentum which is not the case. The uncertainty principle denies it.
$endgroup$
Does this mean that if we want to measure the angular momentum of a particle in state $ψ$, we take $hat L$ and let it act on $ψ$ to give us three possible eigenvalues of $hat L_x$, $hat L_y$ and $hat L_z$, which will correspond to the x,y,z components of the angular momentum?
As you know $L_x$, $L_y$ and $L_z$ are incompatible observables, but note that $L^2$ does commute with the three components of the angular momentum, so:
$$[L^2, Lx] = [L^2, Ly] =[L^2, Lz]=0$$
Which more compactly is:
$$[L^2, L] = 0$$
Based on it, it is feasible to find simultaneous eigenstates of $L^2$ and one of the three components of the angular momentum. Using as an example $L_z$ we would get:
$$L^2 f = lambda f$$
$$L_z f = lambda' f$$
What is the measurement this operator $hat L$ is trying to measure?
Because of compatibility reasons, $L^2$ and the components of the angular momentum are worked out separately.
Actually, to know $hat L$ you should know simultaneously the three components of the angular momentum which is not the case. The uncertainty principle denies it.
edited Dec 20 '18 at 17:53
answered Dec 20 '18 at 17:46


JD_PMJD_PM
15313
15313
add a comment |
add a comment |
$begingroup$
This is right. You cannot have an eigenstate and eigenvalue of this vectorial angular momentum operator $hat{mathbf{L}}$. In fact, one doesn't even need the commutation to see that this cannot work. The reasons are purely mathematical. Just consider that given an operator $hat{O}$, its eigenvalue equation is
$$hat{O} |psirangle = O |psirangle$$
where $O$ is the eigenvalue, which is generally a complex number, but should be a real number for operators representing physical parameters. Now consider what this should look like for vectorial operator $hat{mathbf{L}}$. We should, following the same logic, have it associated with an eigenvalue that is itself a vector, representing a specific angular momentum vector $mathbf{L}$, giving
$$hat{mathbf{L}} |psirangle = mathbf{L} |psirangle$$
But look at the right hand side. We have a vector multiplying a quantum state. That's not mathematically possible. You can only multiply a quantum state by a complex scalar, because that's the only other operation than addition and taking the inner product that is defined on the Hilbert space. So by that token alone, $hat{mathbf{L}}$ cannot have "eigenvalues" in this sense. And this makes sense: the output of applying your $hat{mathbf{L}}$ to a quantum state isn't even another quantum state, but instead the "vector of quantum states" (a "meta-vector", perhaps?)
$$hat{mathbf{L}} |psirangle = (hat{L}_x |psirangle) mathbf{i} + (hat{L}_y |psirangle) mathbf{j} + (hat{L}_z |psirangle) mathbf{k}$$
Thus even worse, technically your proposed "operator" isn't really a proper operator at all, but a map between two rather different vector spaces, and no such maps can have eigenvalues, only self-maps of the same vector space.
$endgroup$
add a comment |
$begingroup$
This is right. You cannot have an eigenstate and eigenvalue of this vectorial angular momentum operator $hat{mathbf{L}}$. In fact, one doesn't even need the commutation to see that this cannot work. The reasons are purely mathematical. Just consider that given an operator $hat{O}$, its eigenvalue equation is
$$hat{O} |psirangle = O |psirangle$$
where $O$ is the eigenvalue, which is generally a complex number, but should be a real number for operators representing physical parameters. Now consider what this should look like for vectorial operator $hat{mathbf{L}}$. We should, following the same logic, have it associated with an eigenvalue that is itself a vector, representing a specific angular momentum vector $mathbf{L}$, giving
$$hat{mathbf{L}} |psirangle = mathbf{L} |psirangle$$
But look at the right hand side. We have a vector multiplying a quantum state. That's not mathematically possible. You can only multiply a quantum state by a complex scalar, because that's the only other operation than addition and taking the inner product that is defined on the Hilbert space. So by that token alone, $hat{mathbf{L}}$ cannot have "eigenvalues" in this sense. And this makes sense: the output of applying your $hat{mathbf{L}}$ to a quantum state isn't even another quantum state, but instead the "vector of quantum states" (a "meta-vector", perhaps?)
$$hat{mathbf{L}} |psirangle = (hat{L}_x |psirangle) mathbf{i} + (hat{L}_y |psirangle) mathbf{j} + (hat{L}_z |psirangle) mathbf{k}$$
Thus even worse, technically your proposed "operator" isn't really a proper operator at all, but a map between two rather different vector spaces, and no such maps can have eigenvalues, only self-maps of the same vector space.
$endgroup$
add a comment |
$begingroup$
This is right. You cannot have an eigenstate and eigenvalue of this vectorial angular momentum operator $hat{mathbf{L}}$. In fact, one doesn't even need the commutation to see that this cannot work. The reasons are purely mathematical. Just consider that given an operator $hat{O}$, its eigenvalue equation is
$$hat{O} |psirangle = O |psirangle$$
where $O$ is the eigenvalue, which is generally a complex number, but should be a real number for operators representing physical parameters. Now consider what this should look like for vectorial operator $hat{mathbf{L}}$. We should, following the same logic, have it associated with an eigenvalue that is itself a vector, representing a specific angular momentum vector $mathbf{L}$, giving
$$hat{mathbf{L}} |psirangle = mathbf{L} |psirangle$$
But look at the right hand side. We have a vector multiplying a quantum state. That's not mathematically possible. You can only multiply a quantum state by a complex scalar, because that's the only other operation than addition and taking the inner product that is defined on the Hilbert space. So by that token alone, $hat{mathbf{L}}$ cannot have "eigenvalues" in this sense. And this makes sense: the output of applying your $hat{mathbf{L}}$ to a quantum state isn't even another quantum state, but instead the "vector of quantum states" (a "meta-vector", perhaps?)
$$hat{mathbf{L}} |psirangle = (hat{L}_x |psirangle) mathbf{i} + (hat{L}_y |psirangle) mathbf{j} + (hat{L}_z |psirangle) mathbf{k}$$
Thus even worse, technically your proposed "operator" isn't really a proper operator at all, but a map between two rather different vector spaces, and no such maps can have eigenvalues, only self-maps of the same vector space.
$endgroup$
This is right. You cannot have an eigenstate and eigenvalue of this vectorial angular momentum operator $hat{mathbf{L}}$. In fact, one doesn't even need the commutation to see that this cannot work. The reasons are purely mathematical. Just consider that given an operator $hat{O}$, its eigenvalue equation is
$$hat{O} |psirangle = O |psirangle$$
where $O$ is the eigenvalue, which is generally a complex number, but should be a real number for operators representing physical parameters. Now consider what this should look like for vectorial operator $hat{mathbf{L}}$. We should, following the same logic, have it associated with an eigenvalue that is itself a vector, representing a specific angular momentum vector $mathbf{L}$, giving
$$hat{mathbf{L}} |psirangle = mathbf{L} |psirangle$$
But look at the right hand side. We have a vector multiplying a quantum state. That's not mathematically possible. You can only multiply a quantum state by a complex scalar, because that's the only other operation than addition and taking the inner product that is defined on the Hilbert space. So by that token alone, $hat{mathbf{L}}$ cannot have "eigenvalues" in this sense. And this makes sense: the output of applying your $hat{mathbf{L}}$ to a quantum state isn't even another quantum state, but instead the "vector of quantum states" (a "meta-vector", perhaps?)
$$hat{mathbf{L}} |psirangle = (hat{L}_x |psirangle) mathbf{i} + (hat{L}_y |psirangle) mathbf{j} + (hat{L}_z |psirangle) mathbf{k}$$
Thus even worse, technically your proposed "operator" isn't really a proper operator at all, but a map between two rather different vector spaces, and no such maps can have eigenvalues, only self-maps of the same vector space.
answered Dec 21 '18 at 1:45
The_SympathizerThe_Sympathizer
3,784923
3,784923
add a comment |
add a comment |
Thanks for contributing an answer to Physics Stack Exchange!
- Please be sure to answer the question. Provide details and share your research!
But avoid …
- Asking for help, clarification, or responding to other answers.
- Making statements based on opinion; back them up with references or personal experience.
Use MathJax to format equations. MathJax reference.
To learn more, see our tips on writing great answers.
Sign up or log in
StackExchange.ready(function () {
StackExchange.helpers.onClickDraftSave('#login-link');
});
Sign up using Google
Sign up using Facebook
Sign up using Email and Password
Post as a guest
Required, but never shown
StackExchange.ready(
function () {
StackExchange.openid.initPostLogin('.new-post-login', 'https%3a%2f%2fphysics.stackexchange.com%2fquestions%2f449543%2fwhat-does-vector-operator-for-angular-momentum-measure%23new-answer', 'question_page');
}
);
Post as a guest
Required, but never shown
Sign up or log in
StackExchange.ready(function () {
StackExchange.helpers.onClickDraftSave('#login-link');
});
Sign up using Google
Sign up using Facebook
Sign up using Email and Password
Post as a guest
Required, but never shown
Sign up or log in
StackExchange.ready(function () {
StackExchange.helpers.onClickDraftSave('#login-link');
});
Sign up using Google
Sign up using Facebook
Sign up using Email and Password
Post as a guest
Required, but never shown
Sign up or log in
StackExchange.ready(function () {
StackExchange.helpers.onClickDraftSave('#login-link');
});
Sign up using Google
Sign up using Facebook
Sign up using Email and Password
Sign up using Google
Sign up using Facebook
Sign up using Email and Password
Post as a guest
Required, but never shown
Required, but never shown
Required, but never shown
Required, but never shown
Required, but never shown
Required, but never shown
Required, but never shown
Required, but never shown
Required, but never shown
WbB4fyslkAqP7jEqMXBNr fh,n3